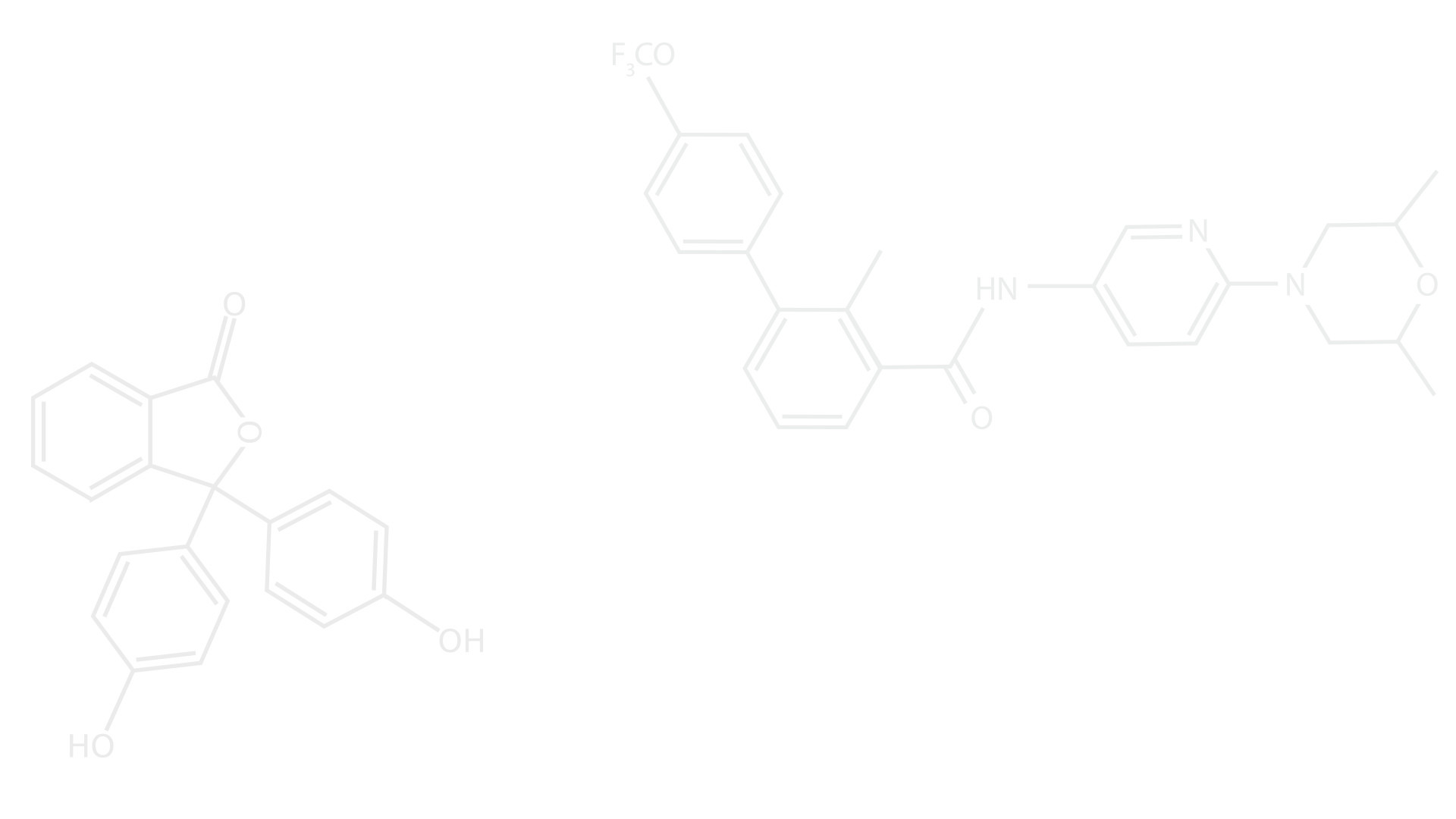
Evaluation of Novel Halide-Based Solid-State Electrolytes for Lithium-Ion Battery Systems
General Overview
Minimizing carbon emissions is critical to reduce the impact of global warming, and renewable energy in the form of solar and wind are already being adopted at exponential rates across the globe to achieve this goal. However, in order for renewable energy infrastructure to fully satisfy the demands of the general population, leaps in battery technologies are required due to the highly fluctuating nature of these power sources, and the implementation of electric vehicles to replace gas-powered gas similarly requires advances in battery technology. High energy density, low cost, and safety are critical factors in choosing the next battery systems that will power our devices.
​
Lithium-ion batteries are already ubiquitous but face limited energy density as well as safety issues with a flammable electrolyte. Replacing the liquid electrolyte with a solid-state electrolyte (SSE) will not only vastly reduce the risk of fire, but it will also yield improvements in energy density and specific capacity. Several classes of SSEs have been investigated, but each comes with its benefits and issues. For example, oxide SSEs offer good ionic conductivity but are mechanically inflexible and prone to fracture during cell assembly, while sulfide SSEs offer very good ionic conductivity at the expense of poor chemical stability.
​
Halide SSEs offer well-rounded benefits, providing good ionic conductivity with appreciable chemical stability when in contact with high-voltage anode and cathode materials, and they have also been shown to remain stable at high voltages. The purpose of this investigation is to synthesize a wide range of halide SSEs primarily using ball-milling with additional post-processing, with the ultimate goal of finding a SSE that shows high ionic conductivity and excellent stability during cycling.
Left: A highly simplified schematic of a battery using a solid-state electrolyte using lithium cobalt oxide (LCO) as the cathode and indium metal as the anode.
Right: A flowchart indicating the current process for SSE synthesis, characterization, and analysis.

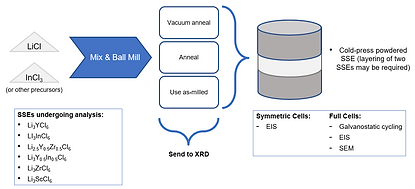
Galvano​static cycling was performed as part of standard battery testing; each full cell is repeatedly charged and discharged at constant current to examine how specific capacity, coulombic efficiency, and other factors change after repeated use. For analyzing ionic conductivity and interfacial resistances, electrochemical impedance spectroscopy (EIS) was employed. X-ray diffraction (XRD) was utilized to confirm that the proper phase has been achieved after each electrolyte batch is synthesized, and scanning electron microscopy (SEM) was employed to examine surface morphology of the electrolyte and its interfaces.
​
A wide range of both well-studied and novel SSEs were successfully synthesized and characterized, with example powders including lithium indium chloride (LIC), lithium yttrium chloride (LYC), and lithium scandium chloride (LSC). LIC and LYC were selected as high-conductivity candidates with excellent stability, with LIC especially stable against high-voltage lithium cobalt oxide (LCO) electrodeposited cathodes employed by the Braun Group.
When employed in full cells, electrodes expand and contract that induce mechanical stress and strain on the cell, which can lead to novel failure mechanisms such as electrolyte loss of contact, void formation, and more, all of which can lead to capacity loss and eventual cell death. These are typically counteracted by cycling cells at high presses (>20 MPa), but this is impractical to scale to applications like smartphone batteries, which for a 10 cm x 4 cm cell would require 8.16 metric tons sitting atop the cell. Low-pressure cycling of solid state batteries is thus a crucial constraint for commercialization.
​
I successfully designed a tunable, low-pressure cell press with improved reliability over previous cell vices in the lab, and I integrated force sensors to measure changes in cell stress in situ. We cycled various cell architectures, ranging from an indium-LYC-LIC-LCO stack to architectures using lithium metal anodes, and found substantial differences in capacity loss across cell architectures and processing methods. This research explored the chemomechanical behavior of all-solid-state batteries and moves the technology one step closer to commercialization.
Enabling stable solid electrolytes for commercial and consumer use will substantially facilitate the renewable energy transition, enabling not only superior consumer electronics but also the grid-scale energy storage solutions necessary for electric vehicles, solar power, and carbon emission reduction.